Key Concepts: Rocks are the record of the environment in which they formed. Different rock types indicate the conditions that existed when they came to be. For sedimentary rocks, these are the environments that existed on the surface of the Earth in the past. Within these rocks, fossils are the remains of ancient living things that existed when those rocks were being proeduced.
The rock record does not merely record environments of the past; it records the fact that those environments have changed radically over the vast scale of deep time. By using the physical relationships between rocks, the changing fossil record, and radiometric means, geologists can reconstruct the age and sequence of events through geologic time. Fossils document a changing panoply of living things over the face of the Earth through this vastness of time.
The Present is the Key to the Past; The Past is Prelude to the Future
Last week we say how we could interpret the environments at the time of formation in different sorts of rocks, and that in the case of sedimentary rocks those particular environments were those on the surface of the planet: the place where atmosphere, ocean, and living this exist. Each rock represents a particular datum. But they can do more than that.
Firstly, the rocks often record that the environment at the time rocks form can be radically different than the environment that exists there now. It is not uncommon to find rocks of the shallow seas on the peaks of mountains (such as Mt. Everest), or the remains of tropical plants in the polar regions, or desert deposits in the middle of England.
Additionally, by looking at the sequence of rocks through time (more about that momentarily) we can see that environments can shift back and forth. Indeed, the geologic record (the sum total of the rocks and the information they contain) were the first indication to humans that environmental change was real, and could be incredibly dramatic. We saw before that we can use observations of the modern world to help us figure out what the environments were like when the rocks form (or, to quote one of the main ideas of geology, "the present is the key to the past"); we will see over the next few weeks how the information of past changes might helps us predict (and survive!) the changes that will happen in the future. This idea is the basic principle behind uniformitarianism: the idea that observations of present conditions help us understand the past, and more broadly that the natural laws of the past were the same as today.
The Rock Cycle: Every Rock is a Record of the Environment in Which it Formed
A major point of modern geoloy: the Rock Cycle:

Rocks (naturally occurring cohesive solids comprised of one or more minerals or mineraloids) are generated in one of three primary manners (which form the basis of rock classification). Or, to put it another way, every rock is a record of the environment in which it formed:
- Igneous
- Formed by the cooling of molten material
- Igneous formed by lava cooling on the surface are volcanic rocks (also called extrusive because they extrude onto the surface)
- Igneous rocks formed by magma cooling at depth are plutonic rocks (also called intrusive because they intrude into previously-existing rocks
- Metamorphic
- Formed by recrystallization of previously existing rocks due to intense heat and/or pressure (i.e., they are baked and/or squashed)
- Will often contain unusual minerals that only form at incredible temperatures and/or pressures
- Sedimentary
- Formed from transported fragments of previously existing rocks
- Bits of previous rock and/or organic matter are called sediment
- Sediment might be in the form of:
- Solid chunks (gravel, sand, silt, mud), which form detrital sedimentary rocks
- Ions in solution, which can
- Be precipitated out as chemical sedimentary rocks
- or be taken up into the living tissues of organisms, made into hard parts like shell or wood, and then deposited when the organism dies to form biogenic sedimentary rocks
- Fossils are common in many sedimentary rocks
- Because they are deposited, sedimentary rocks naturally form horizontal strata.
- Sediment is transported by water, wind, glacial ice, etc.
- Sediments are deposited in deserts, flood plains, rivers, lakes, swamps, coastlines, continental shelves, etc.: depositional environments. The different environments of deposition of sedimentary rocks can preserve different sorts of information in the form of sedimentary structures such as mud cracks, ripple marks, raindrop marks, etc.
- Formed from transported fragments of previously existing rocks
Environments of Deposition
Recall that sedimentary rocks are produced by breaking apart previously-existing rocks and transporting these fragments (as solid sediment or as ions in solution) by wind, water, or ice, to later deposit them (as solid bits of sediment, or as precipitations from solution, or as bits of living things which had absorbed those ions.) The environment in which sediment gets deposited will leave a characteristic set of associations of sedimentary rocks and of sedimentary structures: features left by the sediment by its interaction with the local environment. By observations of modern depositional settings, we can interpret ancient conditions by their sedimentary rocks and sedimentary structures.
It is important to consider, however, that not all environments are environments of deposition. Many locations will be environments of erosion: these places are sources of sediment, but because material is being lost from there rather than accumulating there, they will not wind up in the geologic record. A particular location can shift between deposition and erosion ("D-world" vs. "E-world") as local environmental conditions change.
Here are some aspects of depositional environment to consider:
- Energy of the environment: is it fast-running water, or still water, or wind? The higher the energy, the larger the size of sediment that will be moved around: fast rivers can move cobbles, but slow rivers only silt and mud. If the energy is too high (whitewater rapids, for instance), it will be an erosional rather than depositional setting. For a brief overview of sediment size and the types of energies associated with them:
- Boulders (basically, anything bigger than a baseball): extremely high energy, but short term, events, such as avalanches, tsunamis, etc.; also, glaciers (which move objects slowly but can transport sediment as fine as flour to the size of buildings)
- Cobbles and Pebbles: high energy, sometimes short term (flash floods) but also continuous (the faster moving parts of a stream)
- Sand: moderate energy, such as waves along a beach, the flow of a river channel, or wind in a desert
- Silt: lower energy versions of the same environments as sand
- Mud (such as clay): extremely low energy environments, like lake bottoms, swamps, lagoons, the bottom of the sea, etc.
- Whether the primary sediment source is silicate rocks (such as most igneous or metamorphic rocks) or carbonate minerals (sea shells, carbonate mud, etc.). Most continental (land and freshwater) environments are dominated by silicate sediment deposition, as are marine environments where there is a substantial input from land (e.g., the Atlantic seaboard of North America). If the water is warm, salty (typically), and free of much input of silicate material, then carbonate minerals are likely to be formed: some as chemical precipitates, but far more often in the form of biogenic sediment (broken bits of sea shells or algal skeletons or coral or the like) (e.g., white sand beaches in the Bahamas or the Caribbean).
- The nature of the agent of transportation of sediment.
- Is it wind? If so, the ever-shifting direction of the wind will deposit, uncover, and redeposit the sediment to produce characteristic patterns when you view the sediment (and the rock it becomes) in cross section. These patterns on the side are an example of the sedimentary structure called "cross-beds" (in this case, trough cross-beds).
- Is it wave action (back-and-forth oscillating flow)? This will leave one form of ripples and cross-beds. In contrast, current action (one-directional flow, as in a stream) leaves a different pattern.
- Is it from glaciers? As mentioned above, glaciers simultaneous deposit sediment of all different sizes
- Is it simply settling in quiet water? In that case, only fine particles (clay, silt, etc.) will be present.
- After depositing, was it subjected to:
- Drying? If so, you may find mudcracks.
- Rain? If so, you might (if you are lucky) find preserved raindrop marks.
- Burrowing and mixing by worms, clams, etc. (technically called bioturbation)? You may find the traces of these burrows, but if it goes on enough it might just be a homogenous mixture of sand and silt and clay left over.
- Anoxic (very low oxygen) conditions below the reach of waves or currents? In this case, with no worms or clams to disturb the sediment, the fine layers of clay and silt and so forth will form nice laminations.
- Was the sediment actually a structure built by organisms (like a reef) or a mass of organic material (like plant material (coal) or broken bits of shell)?
By observing modern environments and their sediments and sedimentary structures, we can use the clues mentioned above (as well as other aspects) to reconstruct the paleoenvironment. Major environments of deposition represented in the geologic record include:
- Desert sand dunes
- Debris in front of glaciers
- Swamps
- River channels and their floodplains
- The interiors and margins of lakes
- River deltas
- Reefs
- Lagoons
- Sandy or muddy offshore deposits
- Limy mud from shallow carbonate banks
- Deep sea sediments
- And many, many others
NOTE: Present day sea level is much lower than most of Earth history; also, as new mountains are born, once shallow deposits are uplifted. Consequently, even in the middle of continents, it is common to find sedimentary rocks deposited underwater. In fact, rocks deposited in marine environments are extremely common, even in the interiormost parts of continents.
Whatever the environment of deposition, the sediment is laid down in layers (strata). Since every rock is a record of the environment in which it formed, the strata will be of the same general sort while the environment remains the same, and change to a different sort as the environment changes. Packages of similar strata formed over a region are called formations: at any given spot, if we see a section through the bedrock, we can see the transition from one formation to another, representing a transition from one environment to another.
Lithification and Diagenesis
The above talks about how sedimentary strata are deposited, but it doesn't explain how the loose sediment becomes a cohesive solid (aka a rock). That process is called lithification. Among the primary aspects of lithification are:
- Compaction: as new layers are deposited over older ones, the lower layers get squished, squeezing out ground water in between the sedimentary particles and causing the stratum to compact. In some minerals (clays, for instance), this process may be enough to strongly bind together the sedimentary grains, thus lithifying it.
- Cementation: minerals in the pore water can precipitate between grains of sediment to bind them together.
- Recrystallization: crystals of some minerals (esp. some carbonates) can grow and join together at the pressure and temperature conditions present in burial.
These factors -- alone or in combination -- can bind the sediment together, transforming it into sedimentary rock.
Lithification is an example of diagenesis: post-depositional alteration of sediment. We will see diagenetic effects in another context shortly, in fossilization.
Stratigraphy: Pages of the Book of Time
We saw that sedimentary rocks form by deposition: the settling down of sedimentary particles from suspension in water, ice, or wind. As such, they naturally form layers: strata (singular "stratum"). By observing strata, geologists were able to figure out the sequence of events through time: this is the geologic subdiscipline of stratigraphy.
Early stratigraphers included the Danish polymath Nicolas Steno (1638-1686) and the Scottish geologist James Hutton (1726-1797). Their field observations led to principles we still use in stratigraphy today (which goes to show that sometimes Science is accomplished without the need of expensive complicated equipment.)
Steno's first principle of stratigraphy is incredibly basic: the Principle of Original Horizontality. This is simply the observation that sediment naturally forms horizontal layers when deposited. The reason that this is significant is that due to folding, compression, faulting, and other deformation strata rarely remain horizontal.
Steno's second principle follows from the first: the Principle of Superposition. Unless they have been tilted, the first stratum deposited will be on the bottom, the second oldest deposited on top of that, and so forth. In other words, you go older as you go downwards, and younger as you go upwards. This only gives you the sequence in time, not the amount of time between them; nevertheless, you can now read the local history of the rocks and their environments.
Hutton's first principle is the Principle of Cross-Cutting Relationships: Any structure (folding, tilting, faulting, erosion, igneous intrusion, metamorphism, etc.) which cuts across or otherwise deforms a rock must necessarily be younger than the rock it deforms.
Using these--and some other principles outside the scope of this course--geologists learned how to reconstruct the sequence of events (deposits, intrusions, erosions, folding/tilting, faulting, etc.) for any given location. But how could they correlate these events with those in other regions? If the region wasn't too far, the same rock units (formations) would be present: thus, the rocks that had the same unit of a particular sandstone were deposited when that region had a particular environment present. While this is very effective for a particular region, it won't extend across continents (much less from continent to continent.) Something else was needed.
Index Fossils & the Geologic Time Scale: Chapter Headings for the Book of Time
Fossils--the preserved remains of ancient life--turned out to be useful markers for correlation. The basic pattern had been observed by English geologist and civil engineer William "Strata" Smith (1769-1839) worked extensively in planning and digging canals in Great Britain. He was a keen observer of the rocks and fossils he found. One thing he recognized became his Principle of Fossil Succession. He observed that each fossil species had its own stratigraphic range: the time between the oldest and youngest appearance in the rock record). Consequently, any two rocks that contain the same fossil species must have been deposited between the origin and the extinction of that species.
Not all species were equally good for correlation. Some are so rare they are known from only a single specimen; others lived in only a narrow region. But some are abundant, easily widespread, preserved in many environments, and (most importantly of all) with very narrow stratigraphic ranges. These are termed index fossils, and allowed geologists to correlate over larger and larger distances.
In the 19th Century, geologists began to use index fossils to create a geologic time scale: divisions of ancient time based on the appearance and disappearance of characteristic fossils. The geologic time scale is global in extent: we use the same terms for time for all the continents. Here is the modern (although simplified) version of the whole time scale:
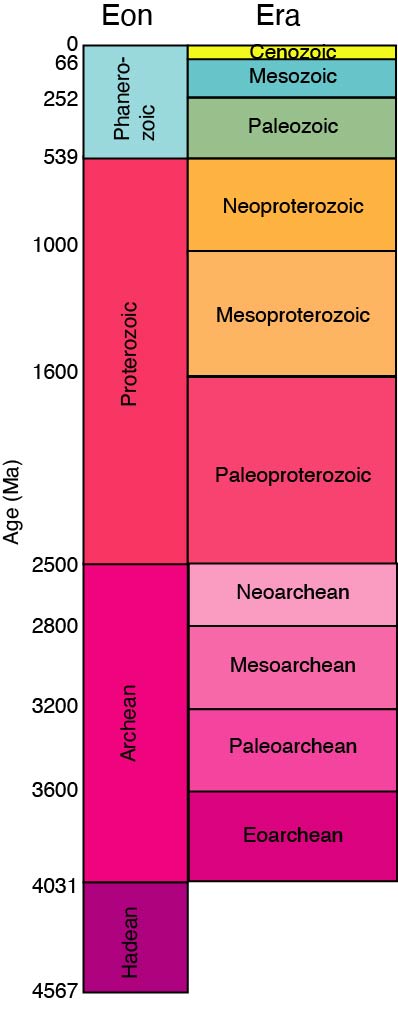
and for the more recent part of Earth History (including most of the history of animal life:
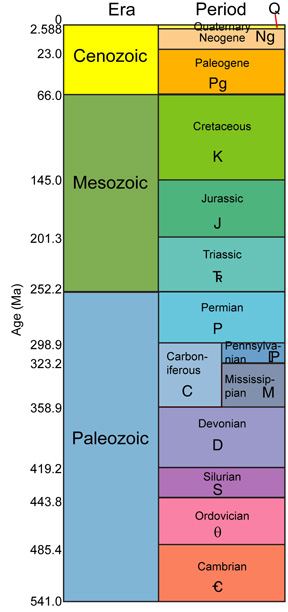
Index fossils gave us a means to divide up geologic time into named segments, but there is a big question they didn't answer: how long ago did these rocks form?
Radiometric Dating: Page Numbers for the Book of Time
The solution to the question of the ages of rock came in the 20th Century, with the discovery of nuclear fission. It was recognized that some naturally-occurring isotopes of elements were radioactive: that is, they decayed into a stable daughter product at a steady rate. Different isotopic series have different decay rates, as discovered in laboratory experiments. When crystals cooled from a melt, they would lock the radioactive atoms into their crystalline structure: as they break down, these decay into their daughter version. A geologist can sample a crystal and measure the ratio of the radioactive parent to the stable daughter product, which gives us an estimate of the number of half-lives that have passed. Multiply the number of half lives by the length of the half life, and you get an estimate on the time since that crystal formed.
(To be fair, the above is a very simplified version that no one does anymore. However, the actual details of the current techniques are outside the scope of the course.)
Radiometric dating of this sort really only works with igneous rocks. However, by using the principles of cross-cutting relationships and superposition, we can at least bracket the ages of sedimentary rocks in between dateable igneous rocks. And by using biostratigraphy (stratigraphy with index fossils) we can correlate estimated age dates from one region to another.
This allowed 20th Century geologists to put numerical dates onto the geologic time scale. And these numbers were vast! Geologists had long suspected that the duration of geologic events were many, many orders of magnitude longer than all of human history. Radiometric dating confirmed this: instead of thousands of years, they were many millions or even billions of years.
In this time scale, entire mountain ranges could be pushed up and then pulverized by erosion and gravity; continents could travel over the globe; seas rise and fall; and dynasties of organisms come and go.
Fossils: Remains of the Life of the World's Past
Humans had long uncovered shells, wood, teeth, bones, footprints, tracks, leaves, and other remains of once-living things preserved in sediments and in sedimentary rock. However, people didn't always recognize these for what they were. After all, how could a once-living thing wind up in solid rock?
Steno worked out how fossils formed. He was one of the first to determine how it was that sedimentary rocks form. Fossils were the remains of living things buried as the sediment accumulated. But what sort of species were represented?
In order to identify fossils, people needed to have some means of recognizing the similarities and differences between species: that is, they needed the science of comparative anatomy. Some of the early comparative anatomists (such as Georges-Louis Leclerc, Comte de Buffon (1707-1788) and Baron Georges Léopold Chrétine Frédéric Dagobert Cuvier (1769-1832)) studied fossils of various sorts.
It was Cuvier in particular who recognized that the anatomy of some fossils actually did resemble modern species, but that many of them were espèces perdues: "lost species". In other words, they were extinct. Extinction was a radical concept at the time. A few species of animals (mostly on islands) had died out in historic time, but few people thought that once-common creatures could utterly disappear from the Earth. But as more and more species from the geologic record were studied, it became apparent that the world had been inhabited in the past by creatures long since vanished.
Cuvier recognized something as well: the implications of Smith's Principle of Fossil Succession. It turned out that each segment of geologic time was inhabited by different assemblages of animals and plants, and the boundaries between these periods saw a large change over in the types of creatures. In the recent geologic past were mammoths and mastodons; before them strange mammals; before them an age of reptiles; and so forth. What explains these divisions in the history of the Earth?
The origin of new forms was the product of evolution by means of natural selection. This doesn't concern ourselves so much in this particular course (take any other course with Holtz & Merck and find out more about it!!) But what about the disappearance of previously existing forms?
Sir Charles Lyell (1797-1875), geologist and popularizer of the idea of uniformitarianism (indeed, the word was coined in reference to him in particular!) thought that the apparent breaks in diversity of life was simply the byproduct of missing time. In his view (and that of his colleague, co-discoverer of Natural Selection Charles Darwin), new forms appear and old ones disappear at gradual rates throughout time. If it appeared that a boundary in the geologic record saw a big change in species, clearly what had really happened is that erosion had removed the intervening time to make it appear as a sudden change. Lyell in particular had a radically extreme view of uniformitarianism, thinking that even rates of change did not vary much in geologic time.
(Infamously, and unlike his colleague Darwin, he didn't accept a genetic connection between species through time. Instead, he thought that species arose and disappeared when conditions favored them, and thus when the same conditions returned now-extinct animals might reign again. Yes, he actually believed that if global warming increased, the world would once again be home to dinosaurs and other currently-fossil reptiles!!)
Alternatively, researchers like Cuvier considered themselves catastrophists. The extreme version of catastrophism believed that natural laws themselves shifted over time, and that observations of the present world had little-to-no bearing on the past. The discoveries of the early geologists and comparative anatomists put paid to this concept, but a smaller-scale version of catastrophism was popular in the early 19th Century. Namely, the idea that the discontinuities in the history of life might represent REAL disasters or crises. We'll see next week that at least this form of catastrophism is in fact real, and that these events inform us (and warn us!) about possible changes in the future.
Mass Extinctions
Above we saw how the world view of Lyell (where major disruptions in the fossil and rock record must have been caused by erosion and missing time) and Cuvier (where such disruptions indicated catastrophes) were in conflict. We actually introduced you to the tools necessary to sort out these differences, but didn't highlight them. In particular, the ability to use radiometric dates eventually allowed stratigraphers to calculate the age of the units below and above the turnovers, and see how far apart in time they were. It was discovered that in at least this context, Cuvier and the catastrophists were correct: these event were rapid, on the scale of million years or less (sometimes many times less).
In the 20th Century came the recognition that these were indeed mass extinctions. Ordinary extinctions seem to happen throughout earth history: in fact, they form what is called "background extinction". But statistical analysis of the appearance and disappearance of forms through time indicated that there were a small number of events in which the loss of species was greatly magnified. Not surprisingly, these fell near or at important geological time boundaries.
In background extinctions the species dying out were often replaced by other species (sometimes close relatives, or even descendants which outcompeted them) which had the same ecological role. But mass extinctions were different: the ecological niches lost sometimes were empty for millions (or tens of millions) of years afterwards.
In order to qualify as a mass extinction, the event had to see:
- Occur over a geologically short period of time
- Extinctions of entire geographically-widespread groups
- Which occupied many distinct different niches and from different parts of the Tree of Life
- Resulting in an overall decrease in Life's diversity for some period of time afterwards.
Paleontologists Jack Sepkoski and Dave Raup did pioneering work in the 1970s assessing rates of extinction in the marine realm over time, and identified the "Big 5" mass extinction events (discussed below). They did recognize that there were some smaller scale disruptions in the history of life as well.
Agents & Mechanisms
Something that is important to distinguish is between the cause (or trigger) behind an extinction event, and the means by which the extinction is actually accomplished.
In particular, we should recognize:
- Causal agents (aka "triggers"): the initial phenomenon that starts the extinction event
- Killing agents: the product of the phenomenon that does the killing. (Note: many causal agents might produce multiple killing agents)
- Killing mechanism: the physiological change produced by the killing agent (e.g., asphyxiation, starvation, heat load; etc.)
In order to identify a reasonable causal agent for any given extinction event, we must first recognize that it would in fact be able to produce killing agents in both the terrestrial and marine realms. It also must not be overkill (after all, EVERY mass extinction event has survivors, and every organism alive today had ancestors that lived through every single mass extinction event so far!). And finally (and most important for this to be more than mere speculation), any proposed causal agent must be identifiable from the rock record independent of the extinction event itself.