The Importance of Paleoclimatology
Paleoclimatology: the study of ancient climates, particularly those before the instrumental record. Paleoclimatologists
study events from the recent past (for example, pre-colonial North America or Australia), but also from the much
more distant past in deep time: the domain of thousands to billions of years ago.
Therefore, we must rely on various proxies for the ancient climate.
Additionally, only by reconstructing past climates can we determine how climate systems operate in the absence of human technological operations.
Furthermore, since written history is only a few thousands of years (and written SCIENTIFIC history only a few centuries at best), there are many natural cycles or ranges of variability of Earth systems which have never played out when humans were making records (or even around).
Climate (the average weather and its variable for a region over time) is the product of a number of factors, most especially:
- The amount of incoming sunlight (mostly a product of the latitude, although also subject to change as the Sun's output varies over time, and the Earth's orbit varies over time)
- The distribution of the heat from this incoming sunlight, affected by:
- The reflectivity or absorbance of the land, ice, or water surface
- The ability of the fluid surface (atmosphere and ocean) to transfer heat
- The degree to which the energy reflected or reemitted from the Earth's surface is trapped by the atmosphere (the greenhouse effect)
None of these features are constant over time, and thus the climate of the world has changed: sometimes quite dramatically. Changes can be produced by:
- Plate tectonics: distribution of the landmasses change; sea levels rise and fall; mountain ranges are built up to redirect prevailing winds; etc.
- Related to this, the large scale ocean currents: redirecting heat to alternately warm up or cool down the planet
- Changes in the abundance and diversity of life, sequestering or releasing greenhouse gases
- Changes in the position of Earth's orbit: a primary driver in wet-and-dry cycles (and during Ice Ages, glacial increase vs. glacial decrease)
- Short term events, like the sudden emission of lots of methane from the sea floor due to volcanism (or of carbons dioxide from one primate species' discovery that burning fossil fuels provides a lot of energy…)
As a result, climates vary over Earth history. On the biggest scale, we see a change between generally hot (greenhouse) conditions with no substantial ice anywhere and generally cold (icehouse) conditions where ice builds up in the mountains and at the poles. The latter can sometimes be shifted further into ice ages, when large glaciers cover much of the continents.
For the geologically recent past, the record of change is much better understood, and we see that climates can shift from one condition to another on a very short period of time. The degree of change of even the geologically recent past can greatly exceed the conditions under which human civilization arose and grew.
The Sedimentary Record of Global Change:
Sedimentary rocks are those formed from the accumulation of fragments of previously-existing rocks. These
fragments might be solid chunks of broken-down rock (i.e., sediment), or ions dissolved in solution, or bits of living
things (which have taken up those ions to build their bodies). These particles then accumulate in different spots
on the Earth (in deserts; in rivers; in the fronts of glaciers; in bays; on the deep sea floor; etc.). Thus, unlike
other types of rock (which form in the depths of the Earth or as molten lava), sedimentary rocks are formed in the
same environments in which organisms live and die, so fossils are common in sedimentary rocks. Very importantly,
since rocks are a record of the environment in which they form, sedimentary rocks are the ones in which
surficial environments are recorded.
Sediment only accumulates in a certain subset of places, called environments of deposition or sedimentary environments:
These are the places in which the particles get washed or blown into and build up. Because of this, some environments
(for example, sea floors) have very good records, while others (for example, mountains and white-water rapids) do not.
Sedimentary rocks can record proxies in a variety of many different forms. They include:
- Particular sedimentary structures (features imposed upon the sediment by physical processes)
- Particular lithologies (rock types)
- Particular physical relationships between lithologies
Some common sedimentary structures include:
- Mud cracks: indicate mud that was wet, then dried, then buried by later sediment
- Ripple marks: indicate
moving wind or water (current in stream; wave action along shore; etc.)
- Symmetrical ripples (aka oscillation ripples) represent water moving back-and-forth, such as at a shoreline
- Asymmetrical ripples (aka current ripples) represents wind or water moving in a single direction
- Crossbeds, aka cross- stratification, are basically the cross-sectional representation of ripple marks, although the tops of the ripples are often lost (truncated) by erosion during the next ripple. Crossbeds are formed by currents (wind or water) pushing sand off the back of a ripple and onto the front (indicate streams, small dunes, etc.)
- Trough crossbeds, cross-section of big sand dunes where shifting winds produced complex movement of sand (deserts, beaches)
- Raindrop marks: indicates a wet surface (but exposed to air) that was rained on, then buried
- Salt casts, representing spots where salt crystals formed from evaporation in an arid environment, but then were dissolved and buried by the return of water
Certain rock types are closely correlated with particular climate conditions:
- Coal requires high plant productivity, and thus indicate wet conditions
- Evaporites ("rock salts") require arid conditions (and thus high rates of evaporation)
- Different forms of soils may indicate arid, temperate, or tropical conditions
- And more
An advantage of sedimentary rocks is that they not only record what happened at a given spot, but they also
record the sequence in which the changes happened. Because sedimentary rocks are deposited out of suspension
or solution, new material is always deposited on top of older material.
However, different depositional environments produce different styles of deposition:
Over time, the strata piles up in big packages that record the changing environment over a given region. |
![]() |
Another source of information in sedimentary rocks are fossils--the preserved remains of
ancient organisms and traces of their behavior preserved in rock. While the vast majority of organisms
wind up inside other organisms (it is what we are built from, and how we run our
metabolisms), a small fraction can wind up being buried in sediment. Once buried, different alterations
may occur: the fossil may be unchanged from the original condition; or it could be permeated with
minerals from groundwater; or it can recrystallize; or it can actually have all its original hard parts
replaced by some other mineral; or more.
While we typically think of fossils in terms of macroscopic organisms (dinosaur skeletons, mammoths frozen in permafrost; petrified wood; leaves; trilobites; etc.), more important for paleoclimate studies are microfossils. These include pollen and spores from plants; certain groups of freshwater and marine single-celled organisms (diatoms, foramiferans, coccolithophorids, etc.); even microscopic crustaceans and insect parts. Fossils can even form sediment themselves. For example, chalk is simply deposits of vast amounts of the skeletons of a single-celled phytoplankton group (coccolithophorids); much of the sea floor is covered in oozes made from phytoplankton and zooplankton; coal is plant matter; etc. |
![]() |
A particular case of fossils as records of past climates are packrat middens. Packrats ( Neotoma cinerea) are common North American rodents which make nests ("middens") by gathering large amounts of debris (mostly plant matter and stones) and urinating on them. Since packrats never wander far from the midden in order to gather their material, these represent good records of what plants were present at a given spot. And since they can be radiocarbon-dated, we can estimate when in time they were formed. So in places like the American Southwest, packrat middens are wonderful sources of information of paleoclimate.
Far more widespread are corals. Corals are calcium carbonate structures built by various species of polyps (basically really tiny sea anemones). The polyps secrete new coral underneath them as they grow outward. This material will reflect the chemical properties in the seawater at the time of formation. Looking down a section of coral will give you a record of changes in sea water at that location.
Speleothems are calcium carbonate deposits in caves: stalactites, stalagmites, and other related structures. Like corals they record changes in environmental condition as they build up.
Glacial ice is yet another form of (highly mobile) sedimentary rock, which forms in layers which give a record of past changes in a number of different ways: amounts of dust; changes in atmosphere trapped in air bubbles; changes in the isotopic composition; etc.
Biostratigraphy
In order to correlated over long distances, something was needed that had a particular non-repeating, unique, global pattern.
William "Strata" Smith, creating first geologic maps of southern England (and expanded out to include the Continent) observed that the pattern of fossils through the strata was consistent from location to location. Developed this into a new stratigraphic principle:
- Principle of Fossil Succession: there is a unique, non-repeating pattern
(history) of fossils through stratigraphic time.
- All rocks containing fossils of the same species were deposited during the duration of that species on Earth.
In order to be an index (or guide) fossil, the organism used must have certain desirable features:
- Have been VERY common, so chances of individuals being buried is good
- Have hard parts, so chances of fossilization are good
- Have a wide geographic range, so that correlation over wide region is possible
- Lived in (or could be deposited in) different environments, so can be found in different formations
- Have some distinctive features, so it can be recognized from closely related forms
- Have a short geological duration (a few million years at most), so finding a fossil of the species in a rock means it had to be deposited in those few million years
The method of using index fossils to correlate rocks is called biostratigraphy. Here is an excellent summary of biostratigraphic correlation.
In combination, the principles of stratigraphy were useful for determining a global relative time scale, but questions of numerical time were still unresolved.
The Geologic Timescale
Using index fossils, geologists were able to correlate across Europe, and then to other continents. During the 19th Century, geologists created a global sequence of events (based on the sequence of (originally mostly European) formations and the succession of fossils) termed the
Geologic Time Scale. Became a "calendar" for events in the ancient past: used to divide up time as well
as rocks.
Geologic Column divided into a series of units: from largest to smallest eons, eras, periods, epochs, ages (or Stages). No initial understanding of the scale of numerical time for these different units.
Animal and plant fossils are mostly restricted to the last (most recent) Phanerozoic
Eon ("visible life eon"). The Phanerozoic Eon is comprised of three Eras:
- The Paleozoic Era ("ancient animal life")
- The Mesozoic Era ("middle animal life"): the "Age of Dinosaurs"
- The Cenozoic Era ("recent animal life"): the "Age of Mammals". We are still in the Cenozoic Era.
Expanding out the Phanerozoic, we can see the different Periods within these three Eras:
We are currently in the Quaternary Period of the Cenozoic Era, and the Holocene Epoch of the Quaternary Period.
No one region has a continuous sequence of time. Any given location has likely had periods of non-deposition or erosion, which would leave gaps in the geological and fossil record at any given spot.
An interactive project on geologic time, for those who want to explore in more detail.
Isotopes and Paleoclimatology:
Isotopes are variations of chemical element with the same number of protons (and electrons) but differ in the number of
neutrons. Isotopes generally have the same properties, but there are some exceptions. For example, some isotopes might be radioactive, while
the others are stable. More importantly for us, some might be favored by certain sets of chemical or physical reactions.
This results in isotope fractionation: starting from an original ratio of different isotopes, a chemical or physical process may preferentially pull one of the isotopes out of the system, resulting in a change in the ratio left in the remaining pool.
A classic example of this is oxygen isotopes in glacial ice. There are two major isotopes of oxygen, 16O and 18O. Evaporation preferentially favors the lighter 16O isotope. Since glaciers are formed from precipitation, their ice has a lower δ18O/δ16O than the water (it is "depleted in 18O" relative to sea water). As glaciers building up, they capture more and more of the 16O, making the oceans progressively enriched in 18O. So:
- During glacial times, sea water's δ18O/δ16O (recorded in marine oozes) goes up as glaciers increase, and it goes down as temperature decreases.
Paleoclimate and Paleogeography:
From a stratigraphic section at single spot, you can get a record of changes over time. From a single moment in time but
multiple localities, you can create a map of paleoclimate. By doing both, one can create paleogeographic climate
maps throughout geologic history.
From these, it has been found that there are three major general states that the world can exist in during the recent part of Earth History (i.e., the last half billion years):
- Greenhouse (or Hothouse) Worlds: times when oceanic currents do not get deflected towards the poles:
- No continental glaciation; very few alpine glaciers; temperatures much hotter than present.
- If there is equatorial circulation, can get deep water generated at the equator: water gets very hot --> evaporation increases --> saltier, denser water sinks. Unlike the modern world, this deep water is warm, and thus contains very little oxygen or carbon dioxide
- Icehouse Worlds: times when oceanic currents are directed towards the poles
- Some continental glaciers; alpine glaciers present; temperatures may still be warmer than present, but cooler than a greenhouse world
- Deep water generated at the poles
- Ice Age World: when an Icehouse world is pushed too far, large continental glaciers form
The Earth has alternated between Greenhouse Worlds and Icehouse Worlds throughout most of the Phanerozoic Eon (the last 542 million years), and plunged into major Ice Age episodes in the Ordovician Period (around 450 Ma), during the Carboniferous and Permian Periods (around 300 Ma), and the Quaternary Period (starting 2.588 Ma). We'll look at that last Ice Age system in much greater detail next week.
Greenhouse of the Cretaceous vs. Icehouse of the Cenozoic:
If we look at a map of the middle part of the Cretaceous Period we see something
very different than today. Today, and in much of Earth history, there is at least some landmass blocking ocean currents
passing through the equatorial regions. During the mid-Cretaceous, however, it was possible to develop a circum-equatorial
current:
- Not all water is directed north or south, to chill in more polar regions
- So a build-up of extremely warm water at the Equator occurs
- High temperature --> high evaptoration --> high salinity
- Increase in salinity causes the water to become denser, so this very warm water plunges down to the bottom of the sea and spreads north and south, warming the water column above it
- Warm water retains less CO2, so the oceans add greenhouse gases into the atmosphere, warming the world, and working as a positive feedback
On the flip side, there is a dramatic temperature drop during the early Cenozoic Era:
- Temperature change occurs extremely fast (less than 100,000 years)
- Drop in bottom water of 4° - 5° C; bigger changes on surface
- Generated by the break up of
Antarctica from Australia, forming circumpolar current and very cold bottom
water
- Some water stays near Antarctica, and thus loses most of its heat.
- Colder water is denser, so eventually this very cold water plunges to the sea floor (the Antarctic Bottom Water), chilling the water above
- Cold water keeps more CO2 than warm water, so the oceans begin to be a sink for greenhouse gases, chilling the world
- This produced the first Antarctic glaciers
- Shift from wet forests to dry woodlands in North America and Europe
Another cooling is produced by the rise of the Himalaya Mountains:
- Rise of a huge mass of rock produced a lot of erosion
- Erosion scrubs CO2 out of the atmosphere, so greenhouse gases go down yet more
- This also causes cooling and drying of the world, leading to the spread of grasslands
An earlier cooling was produced back in the Carboniferous Period of the Paleozoic Era:
- Growth of huge swamps where plants were accumulated and buried in low-lying regions
- This buried plant material becomes coal
- The removal of CO2 out of the atmosphere causes cooling
- It is this coal which is now the major source of anthropogenic greenhouse gases!
The Paleocene-Eocene thermal maximum (PETM) was a very short term global warming event 55.8 Ma in which for a geologically-short period of time (<10,000 years) the level of CO2 jumped to 3-4x the previous background level. (This jump is comparable to the upper end of anthropogenic greenhouse gas models, but from a higher starting point.) PETM saw a temperature increase of +4-5K at the tropics, +6-8K at the poles, and even +4-5 K in the deep sea.
Some consequences of this event:
- Extinction of many species of plankton, and of additional sea and land organisms
- A jump of ~3x background level of the amount of insect damage seen on leaves, lasting for about 10 kyr
- Short term (~a few ky) dwarfing of terrestrial animals, probably as a result of environmental stress
- Major changes in the habitat ranges of species: in particular, warming polar regions allowed the spread of subtropical and tropical animals from continent to continent over corridors which were once too cold for them to enter
The likely cause of PETM was due to a massive release of methane clathrates from the sea floor. (This matches the isotopic signature of the carbon increase.) In turn, this is likely due to a sudden burst of submarine volcanism in the nort
Ice Ages:
Since the early 1800s geologists noticed glacial-type landforms (erratic boulders, striations, outwash, etc.) in regions
far from mountains in Europe and North America. This led some to recognize the existence of Ice Ages.
The Cretaceous and early Paleogene was a greenhouse world. From the Late Paleogene onward, we have been in an ice house conditions occurred during that interval. During last 2.6 million years (the Quaternary Period), the situation has become extreme, an ice age with major continental glaciations alternating with interglacials. The interval from 2.588 Ma to 10,000 years ago is called the Pleistocene Epoch. The last interglacial of the Quaternary Period (the one we are in) is the Holocene Epoch.
Pleistocene temperature and Proxy Data: The first reliable thermometers went into use in Italy in the late 17th century. The first continuous records of daily temperature didn't begin until the early 19th century in England, so how do we know about ancient temperatures? We infer them indirectly through proxy data:
- Glacial landforms: document the presence of glaciers, but are a very blunt instrument. The problem with glaciers is that they don't record temperature precisely and that new ones bulldoze away the traces of older ones. As a result, ancient moraines and such only tell us so much about the history of glacial intervals.
- Geochemical data gives a clearer picture.
The Oxygen isotope record: we saw above that we can reconstruct the ocean's isotopic history by looking at the ratio of oxygen isotopes present in foraminiferan shells deposited at different times. That ratio, in turn, tells us how much water was locked up as continental ice.
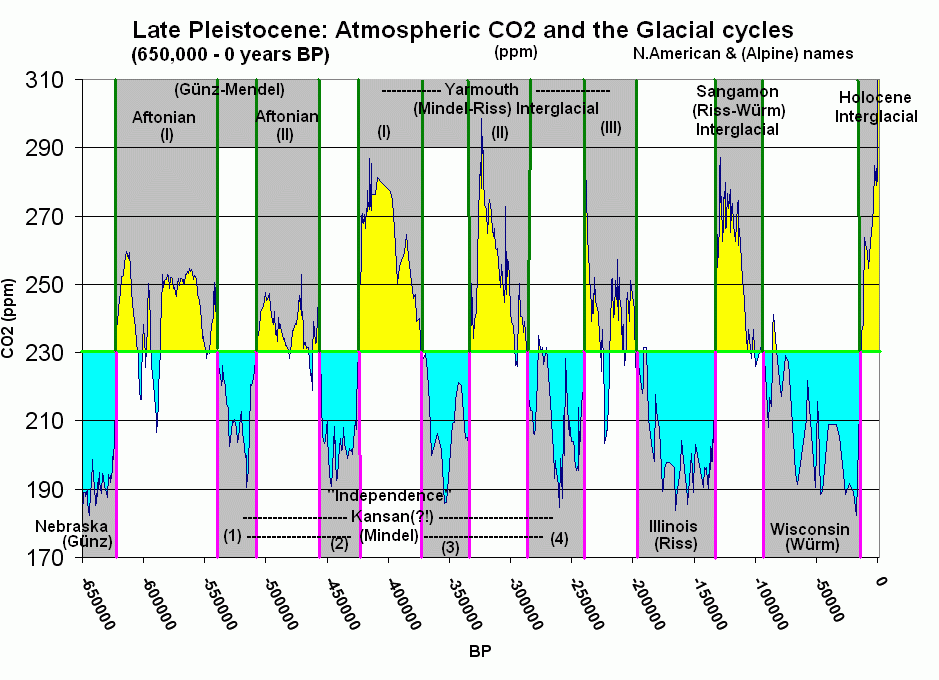
Apparently there were closer to thirty distinct glacials and interglacials. On a longer time scale,
we see that these cycles have gradually increased in severity.
Global Cooling and Ice Ages:
Definitions:
- Glacial: Interval of intense and extensive continental glaciations, characterized by dramatic climate fluctuations.
- Interglacial: Interval of relatively constant warm climate with lesser continental glaciation.
- Ice Age: Interval characterized by the prolonged alternation of glacials and interglacials.
- Milankovitch cycles: In the 1920s, the Yugoslavian meteorologist Milutin Milankovitch
realized The Earth's movement through space is subject to three kinds of cycles:
- Orbital eccentricity: The orbit around the Sun is an ellipse that changes shape (becoming more and less
circular) in a cycle of 100,000 years.
- Axial inclination: The axis of rotation is tilted. The angle of tilt varies from 21.5 deg. to 24.5 deg. in a cycle of 41,000.
- Axial precession: The axis of rotation wobbles around an axis like that of a toy top. So, today the axis points toward Polaris, the north star, but in earlier times, it didn't. One full precessional wobble takes 23,000 years.
- Orbital eccentricity: The orbit around the Sun is an ellipse that changes shape (becoming more and less
circular) in a cycle of 100,000 years.
Solar forcing: The sum of the effects of these cycles gives the general tendency for glaciers to form. Note: Solar forcings are different at different latitudes and in different hemispheres.
Factors Influencing the growth of continental glaciers:
- Mild summers: Each cycle influences the severity of winters to some degree, but what really matters is
the mildness of summers. It snows hard in many places. Glaciers only form in regions where at least some of the
previous winter's snow survives the summer. Thus, when Milankovitch cycles favor mild summers, as when:
- orbital eccentricity is great,
- axial inclination is slight,
- axial precession is such that summer comes when Earth is farthest from the sun,
- Ice-house world conditions prevail: The slight differences in summer weather caused by Milankovitch
cycles doesn't make a lick of difference in greenhouse world conditions in which no winter snow has a prayer of
surviving the summer. Thus, ocean circulation must bring water to the poles before we can think about having
glaciations.
- Large land masses (snow-catchers) exist in high latitudes Consider past ice ages:
- Quaternary Period:, The northern continents are close to the North Pole and Antarctica is at the South Pole.
- Carboniferous Period: The southern half of Pangea lay over the South Pole.
- Late Proterozoic Snowball-Earth episode: Not well understood but all indications are that the supercontinent of Rodinia blocked circumtropical circulation and extended into high latitudes in both hemispheres. Remember, this was when continental glaciers extended into the tropics, and the majority of the ocean was covered in sea ice.