Cutaway of the Earth’s interior. The abundance of seismic data from numerous seismometers around the globe allows us to investigate detailed structure of the interior.
A key seismic tool for unraveling the origins of lateral heterogeneity inside the Earth comes from studying the depth and sharpness of abrupt discontinuous changes in elastic properties over depth. These boundaries, known as seismic discontinuities, delineate the major vertical stratification within the Earth, including the Mohorovičić discontinuity at the base of the crust, the transition from solid mantle to molten outer core at the Core Mantle Boundary, and the Inner/Outer Core Boundary at the solid metallic core. Aside from this major stratification in seismic properties, there are a variety of secondary discontinuities within the mantle, arising from a wide range of mechanisms, including changes in mineralogy, state, composition, melt content, anisotropy or a combination of the above.
The terrestrial component of research in our group centers on the seismological determination of the depth and sharpness of elastic contrasts that arise from high-pressure and temperature solid-to-solid phase changes in minerals, rheological transitions in material properties, the presence (or absence) of melt, and the chemical differentiation and stratification inside the Earth. We are developing multiple techniques for using large, high-quality, broadband, seismological array recordings of earthquakes to amplify low amplitude signals out of the background noise, both from existing datasets and new datasets collected in temporary field deployments of seismic arrays.
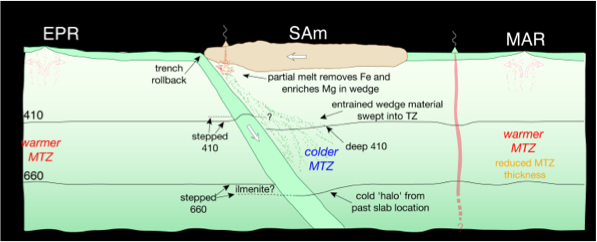
Our research brings together results from both new and established seismic techniques, providing an integrated and detailed image of discontinuity structure beneath well sampled regions, a result crucial for answering questions about the dynamics and evolution of Earth’s interior. Some of our research targets include the nature of the lithosphere-asthenosphere boundary, the lateral existence and character of “X” discontinuities, the global topography, sharpness, and impedance contrast of the 410 and 660 km discontinuities of the mantle, and intermittent boundaries, such as the 1000 km discontinuity, the 520, and the Lehmann.
The raypath geometry of the SS precursor seismic phase.
One particularly useful set of seismic phases for interrogating mantle structure are the SS precursors. These seismic body waves arrive several hundred seconds before their parent SS phase, which reflects off the crust at the center of its path. The precursors reflect from deeper interfaces, and are sensitive to discontinuity structure far away from sources and receivers, making them ideal for imaging the remotest places on the Earth. I have focused on using these phases to image many of the features listed above, and used the precursors to find new discontinuities in the Earth’s mantle. Our group is investigating other seismic phases that are sensitive to mantle structure and developing new algorithms for recovering Earth structure using seismology.
Variation in the depth of the upper mantle discontinuities from thermal and chemical heterogeneity.
Relevant Work:
- Schmerr, N., Imaging Mantle Heterogeneity with Upper Mantle Seismic Discontinuities, chapter in Mantle Heterogeneities, edited by. Khan, A. (2015) http://dx.doi.org/10.1007/978-3-319-15627-9_3.
- Huang, Q., Schmerr, N., Waszek, L., and Beghein, C., (2019), Constraints on Seismic Anisotropy in the Mantle Transition Zone from Long-Period SS Precursors. Journal of Geophysical Research – Solid Earth, https://doi.org/10.1029/2019JB017307.
- Waszek, L., Schmerr, N., Ballmer, M., (2018) Global observations of reflectors in the mid-mantle with implications for mantle structure and dynamics. Nature Communications, 385 http://dx.doi.org/10.1038/s41467-017-02709-4.
- Whittaker, S.^, Thorne, M. S., Schmerr, N. C. and Miyagi, L. (2016), Seismic array constraints on the D″ discontinuity beneath Central America. J Geophys Res-Sol Earth http://dx.doi.org/10.1002/2015JB012392.
- Auer, L., Becker, T. W., Boschi, L. and Schmerr, N. (2015), Thermal structure, radial anisotropy, and dynamics of oceanic boundary layers. Geophys Res Lett 42, 9740–9749 http://onlinelibrary.wiley.com/doi/10.1002/2015GL066246/full.
- Ballmer, M., Schmerr, N., Nakagawa, T., Ritsema, J. (2015), Compositional mantle layering revealed by slab stagnation at ~1,000 km depth, Science Advances, 1, e1500815–e1500815, http://advances.sciencemag.org/cgi/doi/10.1126/sciadv.1500815.
- Rader, E., E. Emry, N. Schmerr, D. Frost, C. Cheng, J. Menard, C.-Q. Yu, and D. Geist (2015), Characterization and petrological constraints of the mid-lithospheric discontinuity, Geochemistry, Geophysics, Geosystems, http://dx.doi.org/10.1002/2015GC005943.
- Lessing, S., C. Thomas, M. Saki, N. Schmerr, and E. Vanacore (2015), On the difficulties of detecting PP precursors, Geophysical Journal International, 201(3), 1666-1681 http://dx.doi.org/10.1093/gji/ggv105.
- Reeves, Z.^, V. Lekic, N. Schmerr, M. Kohler, and D. Weeraratne (2015), Lithospheric structure across the California Continental Borderland from receiver functions, Geochemistry Geophysics Geosystems, 16(1), 246-266 http://dx.doi.org/10.1002/2014gc005617.
- Zhao, C., E. J. Garnero, A. K. McNamara, N. Schmerr, and R. W. Carlson (2015), Seismic evidence for a chemically distinct thermochemical reservoir in Earth's deep mantle beneath Hawaii, Earth and Planetary Science Letters, 426, 143-153 http://dx.doi.org/10.1016/j.epsl.2015.06.012.
- Beghein, C., K. Yuan, N. Schmerr, and Z. Xing (2014), Changes in Seismic Anisotropy Shed Light on the Nature of the Gutenberg Discontinuity, Science, 343(6176), 1237-1240 http://dx.doi.org/10.1126/science.1246724.
- Porter, R. C., M. J. Fouch, and N. C. Schmerr (2014), Dynamic lithosphere within the Great Basin, Geochemistry Geophysics Geosystems, 15(4), 1128-1146 http://dx.doi.org/10.1002/2013gc005151.
- Rychert, C. A., N. Harmon, and N. Schmerr (2014), Synthetic waveform modelling of SS precursors from anisotropic upper-mantle discontinuities, Geophysical Journal International, 196(3), 1694-1705 http://dx.doi.org/10.1093/gji/ggt474.
- Schmerr, N. C., B. M. Kelly, and M. S. Thorne (2013), Broadband array observations of the 300 km seismic discontinuity, Geophysical Research Letters, 40(5), 841-846 http://dx.doi.org/10.1002/grl.50257.
- Long, M. D., C. B. Till, K. A. Druken, R. W. Carlson, L. S. Wagner, M. J. Fouch, D. E. James, T. L. Grove, N. Schmerr, and C. Kincaid (2012), Mantle dynamics beneath the Pacific Northwest and the generation of voluminous back-arc volcanism, Geochemistry Geophysics Geosystems, 13 http://dx.doi.org/10.1029/2012gc004189.
- Rychert, C. A., N. Schmerr, and N. Harmon (2012), The Pacific lithosphere-asthenosphere boundary: Seismic imaging and anisotropic constraints from SS waveforms, Geochemistry Geophysics Geosystems, 13 http://dx.doi.org/10.1029/2012gc004194.
- Schmerr, N. (2012), The Gutenberg Discontinuity: Melt at the Lithosphere-Asthenosphere Boundary, Science, 335(6075), 1480-1483 http://dx.doi.org/10.1126/science.1215433.
- Schmerr, N., and C. Thomas (2011), Subducted lithosphere beneath the Kuriles from migration of PP precursors, Earth and Planetary Science Letters, 311(1-2), 101-111 http://dx.doi.org/10.1016/j.epsl.2011.09.002.
- Schmerr, N., E. Garnero, and A. McNamara (2010), Deep mantle plumes and convective upwelling beneath the Pacific Ocean, Earth and Planetary Science Letters, 294(1-2), 143-151 http://dx.doi.org/10.1016/j.epsl.2010.03.014.
- Schmerr, N., and E. J. Garnero (2007), Upper mantle discontinuity topography from thermal and chemical heterogeneity, Science, 318(5850), 623-626 http://dx.doi.org/10.1126/science.1145962.
- Schmerr, N., and E. Garnero (2006), Investigation of upper mantle discontinuity structure beneath the central Pacific using SS precursors, Journal of Geophysical Research-Solid Earth, 111(B8) http://dx.doi.org/10.1029/2005jb004197.